In my previous post, I summarized a major test success and milestone at Applied Ion Systems with the successful use of the micro pendulum test stand to gather impulse bit data from the pulsed plasma thrusters being researched and developed through these efforts. The full report can be found in the newly added Reports page, where I will post every test report for each test run at Applied Ion Systems – fully open source data, analysis, and results, regardless of success or failure! This one however, was a major success! The report can also be downloaded here as well:
To measure impulse bit, several key pieces of information need to be known about the pendulum. If the pendulum mass and length is known, and based on the displacement of the pendulum, force on the pendulum can be calculated. The following equation is a simple way of determining this:
Ibit = m* √ (2*g*(L- √ (L^2-x^2)))
Where:
- Ibit = Impulse Bit (Ns)
- m = pendulum mass (kg)
- g = acceleration due to gravity (m/s^2)
- L = pendulum length (m)
- x = pendulum displacement (m)
The entire test was captured on camera, with the pendulum aligned vertically and the camera centered. For each shot, the video was manually stepped and paused at the point of maximum displacement, and lines overlaid to calculate the angle. Since the length of the pendulum is known, and the angle of displacement now found, total displacement can be calculated through simple trigonometry. Using this displacement, impulse bit could be estimated. Some examples of a few test shots can be seen below with captured angles:
The following table shows all of the data from the impulse bit test:
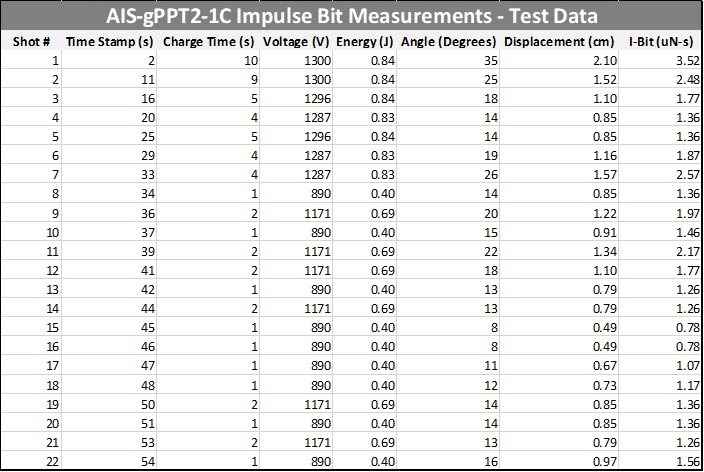
In all, 22 shots were recorded, over varying energies. Charging voltage was estimated based on the time interval between shots, as well as the main capacitor value, and charging supply characteristics. From this, stored energy for each shot was estimated. Charging voltages ranged from 890V to 1300V, corresponding to energies ranging from 0.40J to 0.84J respectively. Based on the measured angle, displacement can be calculated, and from this, with known pendulum parameters, impulse bit can be estimated.
A wide range of impulse bits were recorded from the test. The results are plotted below, comparing impulse bit to stored energy in the primary capacitor:
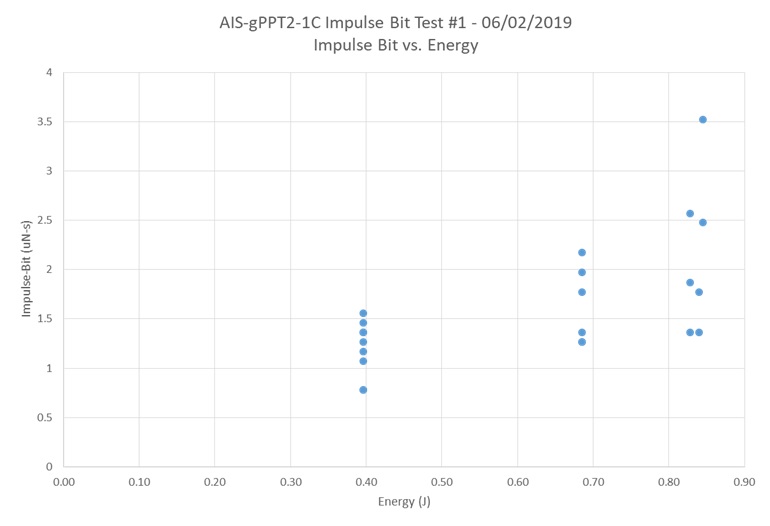
Impulse bit ranged from 0.78uN-s to 3.52uN-s. An interesting trend can be observed from the data for this particular thruster – as capacitor energy increases, so does the shot-to-shot variation for recorded impulse bit. However, as a whole, impulse bit increases as energy increases, as expected. By looking at the average impulse bit for each energy tested, we get the following numbers:
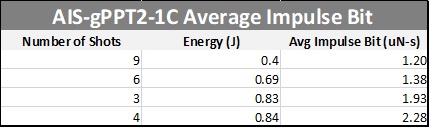
Plotted, we can see the following trend:
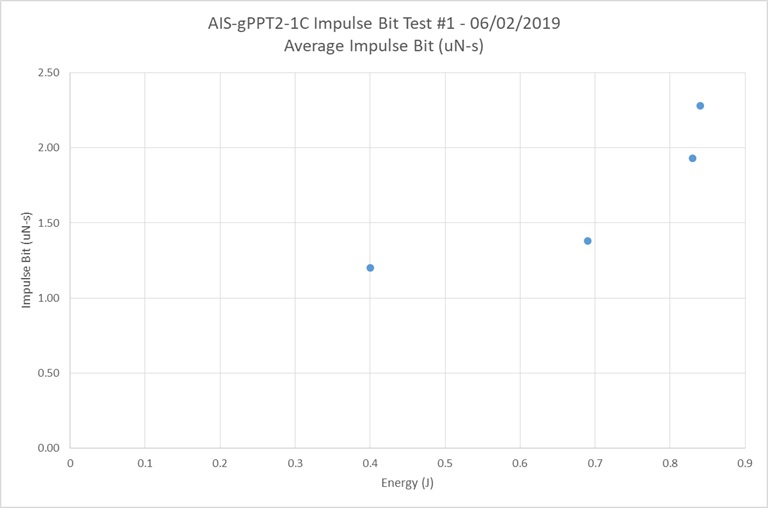
In literature, while larger electromagnetic (EM) dominated PPTs follow relatively linear scaling trends for impulse bit vs. energy, coaxial electrothermal (ET) thrusters, especially in the lower-energy realm (10 Joules or less), shows much larger shot-to-hot variation, as well as non-linear scaling, which can also be observed n these test results as well. Based on the limited data available for sub-Joule pulsed plasma thrusters, performance is within the expected range for such a small PPT. For future tests, more data points will need to be collected and compared, however ultimately for this to be accomplished, thruster lifetime must be significantly improved.
In addition, based on the predicted max allotted power for a 1P Pocketqube for propulsion, it is expected that the max repetition rate of such a thruster would be on the order of 0.25-0.33 Hz (though slightly higher may be achieved at lower energies still), allowing for predicted thrust of around 0.5uN.
As of now, designs are already complete for the next generation thruster of the gPPT series – the AIS-gPPT3-1C thruster. This thruster aims to greatly improve performance of its predecessor through several key changes. First, available fuel is greatly increased by reducing the fuel bore diameter from 0.15″ down to 0.0625″. In addition, the fuel bore is lengthened from 0.125″ to 0.1875″. As the thruster fires, material ablates, widening the bore. Eventually, enough material will ablate that the thruster will no longer operate effectively. The current limit for the AIS-gPPT2-1C thruster is estimated to be only 500 shots. A narrower and longer starting bore has the advantage of greatly increasing the amount of available fuel, while keeping the form factor tight and small. Total thruster length has only increased by 0.0625″ in the newest iteration.
A second major change with the new thruster design is the incorporation of a magnetic nozzle in the anode. By using a magnetic nozzle, the plasma plume can be focused, and much like a classic converging-diverging nozle, magnetic nozzles can contribute to performance by increasing plasma acceleration.
Finally, the newest thruster design will explore 4 fuels, three of which have most likely never been tested before, or at least data reported, for PPTs – PEEK, Ultem, and a unique Bismuth-Tin fuel, in addition to standard Teflon fuel. The goal of these new fuels will be to primarily explore improved lifetime of this tiny PPT by using materials with higher ablation resistance than standard Teflon. While this will decrease impulse-bit and thrust, specific impulse and lifetime should increase. For such a small thruster with limited fuel and space, lifetime is key to successful implementation for something like a Pocketqube-sized satellite.
This is the first data set collected for thrusters developed at Applied Ion Systems, and has provided a valuable first insight to the performance of these tiny thrusters. Going forward, the next versions will provide more data, and major improvements can be made. Lots of new and exciting developments to come!